Professor Bill Harris FRS
01223 333772
Professor of Physiology, Development and Neuroscience
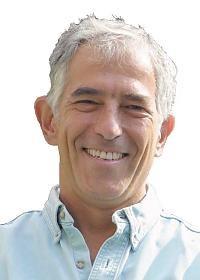
EPSRC CDT in Sensor Technologies for a Healthy and Sustainable Future
Sensor CDT
Department of Chemical Engineering and Biotechnology
Philippa Fawcett Drive
Cambridge
CB3 0AS
© 2024 University of Cambridge